In 2018, Illinois researchers were among the first to experimentally demonstrate a new phase of matter called a quadrupole topological insulator — a unique species of material that can lend a helping hand in the search for new quantum technologies. Now, several years after the initial discovery, they and collaborators in Germany have successfully downsized the technology to smaller than the width of a human hair, without losing its unique properties.
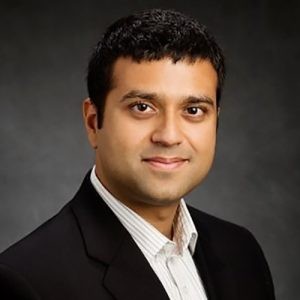
Gaurav Bahl, professor of mechanical science and engineering at the University of Illinois, participated in both the initial discovery and the recent work. The experiments showing the dramatic improvement were carried out TU Kaiserlautern in Germany under the guidance of Georg von Freymann, professor of experimental physics. The work is published recently in the journal Nature Communications.
Topological insulators are unique materials that exhibit protected conductive states along their boundaries, despite being insulating on the inside. This special property allows their states to be “robust”—easier to control and harder to disrupt—which is a valuable characteristic for many quantum technologies. Quadrupole topological insulators (QTIs) are even more unique, where the excitations in the material can arrange into zero-dimensional point states. This widens the range of potential applications accessible to topological insulators.
“When you or I sketch out a device on a sheet of paper, we typically need to be able to draw lines and dots,” Bahl said. “QTIs give us the dots we have always needed to make really robust devices. Our original demonstration of the QTIs used circuit boards connected up in a tricky configuration, and the resulting point states were composed of microwave resonances. While this demonstrated the physics concept, it wasn’t really of much practical use. The current work is a huge step towards nanoscale QTIs for photons, which are individual particles of light that are often used as quintessential quantum information carriers.”
The researchers accomplished their new demonstration by making a photonic “synthetic material”—a model of a material, with “atoms” connected by “bonds”. The atoms are represented by individual photonic waveguides, tubes that carry light, whose electromagnetic properties resemble electronic states in atoms; the bonds are represented by the overlap of the electromagnetic fields of these waveguides, similar to how electrons for bonds by interacting in a material. Essential to this work, the researchers also engineered the shape of these waveguides to make their electromagnetic fields emulate well-known electron orbitals.