Find the full series here or browse the article's below.
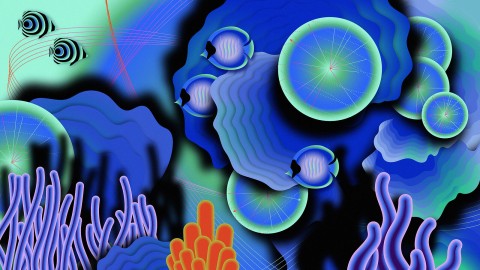
Building the quantum workforce
Published on 02/01/22
Written by Amanda Solliday
Engineers, physicists, computer scientists and more are needed for the second quantum revolution.
For most of us, quantum computing, next-generation quantum sensing and quantum networking still belong to the future. But many early-career scientists and students are already preparing for that future.
Physicist Reina Maruyama, who studies neutrinos and dark matter at Yale University, says she has seen a flood of students and postdocs interested in quantum information science.
To Maruyama, this buzz is promising news. “When there is an infusion of new people and new ideas, there's likely to be a big advance in technology,” she says. “I’m excited about that, so then I can combine this new technology with really exciting science.”
Making strides in quantum information science and its applications will require cooperation among experts from a variety of backgrounds, she says.
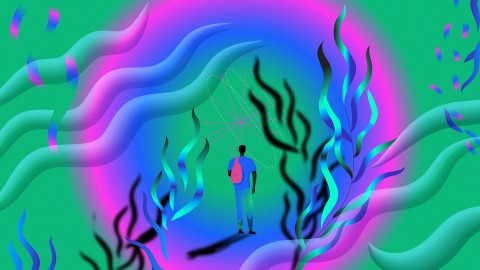
#BlackInQuantum
Published on 01/27/22
Written by R.M. Davis
Black scientists have played important roles in the history of quantum physics. In this growing area of specialization, there’s an opportunity to continue that legacy.
The sixth person to earn a doctoral degree in physics from an American institution was African American scientist Edward Alexander Bouchet. It was 1876, just 11 years after the US had adopted a constitutional amendment that outlawed slavery.
Never again have African Americans made up such a large percentage of US physicists. In fact, it would take another 42 years before a second African American man earned a physics PhD in the United States.
That was Elmer Imes, a contemporary of Albert Einstein, who earned his degree from the University of Michigan in 1918. His doctoral work established some of the earliest direct experimental evidence of quantum theory, making him a crucial historical figure in quantum physics and quantum information science.
But the accomplishments of Black scientists like Imes and Bouchet are not well known, says Charles Brown, a postdoctoral researcher at the University of California, Berkeley. “Black people have been contributing to the progress of research groups for a long time, and just not really having enough of a spotlight on them… which is why when you go to Wikipedia, and you try to look people up, it's hard to find anything,” he says.
Like Imes, Brown searches for insights into the quantum realm. And like fellow Yale graduate Bouchet, Brown finds himself in a small pool of physics PhDs: According to a survey by the American Institute of Physics, “quantum foundations and information science” ranks among the smallest subfields in physics, having granted an average of just 30 PhDs per year in 2017 and 2018.
The US and other nations plan to grow what they’re calling the “quantum workforce,” and they’re going to need people with the right level of expertise to fill it, says Thomas Searles, an associate professor at the University of Illinois, Chicago. “There is a large issue of this new, burgeoning industry where there are going to be significant problems with the workforce and sustaining it,” he says.
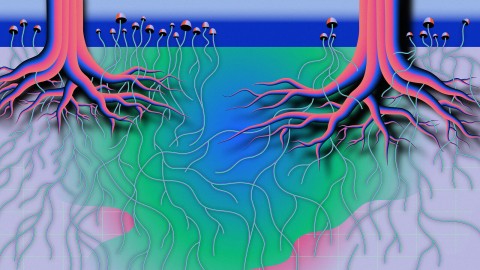
What is a quantum network?
Published on 01/26/22
Written by Mara Johnson-Groh
As we step into the quantum age, here are four things to know about quantum networks.
Four years, four months, and twelve days ago, a photon—a particle of light—left Proxima Centauri, the closest star to us. Just now, it finally arrived at Earth.
This photon, and others that have come with it, could reveal incredible secrets about the planets that orbit the red dwarf star—such as if they’re habitable, or even inhabited. However, with current instruments, we’re not able to tease out this information.
That could one day change with technology called quantum networks.
Quantum networks are like the classical networks we use in everyday life to transmit and share digital information. However, quantum networks use quantum bits, or qubits, which encode information in a way that is utterly foreign to the classical way of thinking. Qubits use tricks from the weird world of quantum mechanics and are fundamentally different from classical computing bits. And when employed on quantum networks, they are radically more powerful.
Quantum networks don’t exist—and many scientists in the field will tell you they’re a long way off. But when they arrive, they could revolutionize everyday life, making unhackable communications secured for banking, medicine, navigation and more. We might not be there yet, but already scientists are testing the building blocks and putting together prototype systems.
“There are breakthroughs happening all the time,” says Sophia Economou, a physics professor and quantum information expert at Virginia Tech.
Already, basic quantum communications called quantum key distributions are helping secure transmissions made over short distances. But before quantum networks become commonplace, they’ll likely make their more public debut in scientific settings.
As we step into the quantum age, here are four things to know about quantum networks.

The quantum squeeze
Published on 01/25/22
Written by Evelyn Lamb
A technique from the newest generation of quantum sensors is helping scientists to use the limitations of the Heisenberg uncertainty principle to their advantage.
In the 1960s, researchers at the science lab of the Ford Motor Company developed the superconducting quantum interference device, also known as a “SQUID.” It was the first usable sensor to take advantage of a quantum mechanical property—in this case, superconductivity.
That made the SQUID one of the first generation of quantum sensors: devices that use a quantum system, quantum properties or quantum phenomena to make a physical measurement. Physicists took the idea and ran with it, coming up with new types of sensors they continue to use and improve today.
SQUIDs have played a key role in the development of ultrasensitive electric and magnetic measurement systems and are still in use. For example, they amplify the detector signals for the Super Cryogenic Dark Matter Search. “As particle physicists, we’ve been using quantum sensing techniques for decades,” says SuperCDMS physicist Lauren Hsu of the US Department of Energy’s Fermi National Accelerator Laboratory.
But SQUIDs are no longer the only quantum sensors around. One important recent development in quantum sensing is known as quantum squeezing—a way to circumvent quantum limitations that even quantum sensors have faced in the past.
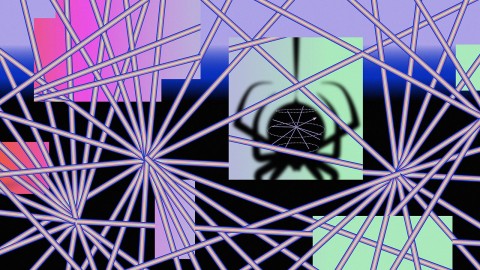
Going beyond the exascale
Published on 01/20/22
Written by Emily Ayshford
Quantum computers could enable physicists to tackle questions even the most powerful computers cannot handle.
After years of speculation, quantum computing is here—sort of.
Physicists are beginning to consider how quantum computing could provide answers to the deepest questions in the field. But most aren’t getting caught up in the hype. Instead, they are taking what for them is a familiar tack—planning for a future that is still decades out, while making room for pivots, turns and potential breakthroughs along the way.
“When we’re working on building a new particle collider, that sort of project can take 40 years,” says Hank Lamm, an associate scientist at the US Department of Energy’s Fermi National Accelerator Laboratory. “This is on the same timeline. I hope to start seeing quantum computing provide big answers for particle physics before I die. But that doesn’t mean there isn’t interesting physics to do along the way.”
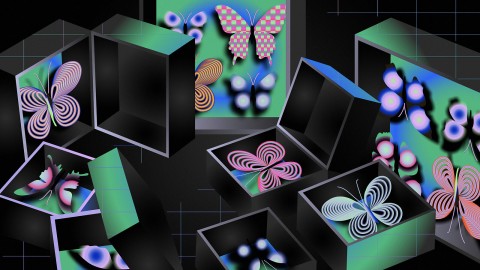
More than one way to make a qubit
Published on 01/19/22
Written by Christopher Crockett
Scientists are exploring a variety of ways to make quantum bits. We may not need to settle on a single one.
The goal of building a quantum computer is to harness the quirks of quantum physics to solve certain problems far faster than a traditional computer can. And at the heart of a quantum computer is the quantum bit, or qubit—the quantum equivalent of the 1s and 0s that underlie our digital lives.
“A qubit is the fundamental building block of quantum information science technology,” says Joseph Heremans, an electrical engineer at the US Department of Energy’s Argonne National Laboratory.
Traditional bits can be any sort of switch, anything that can flip from 0 to 1. But building a qubit takes something more.
“A qubit is essentially a quantum state of matter,” Heremans says. “And it has weird properties that allow you to store more information and process more information” than a traditional bit.
Those weird properties include superposition (the ability to be in a mixed state, a weighted combination of 1 and 0) and entanglement (in which multiple qubits share a common quantum state). Both might seem like they’d be hard to come by. Fortunately, nature has provided lots of options, and engineers have cooked up a couple more.
Researchers are exploring more than half a dozen ways to implement qubits, with two promising approaches currently in focus: superconducting circuits and trapped ions.
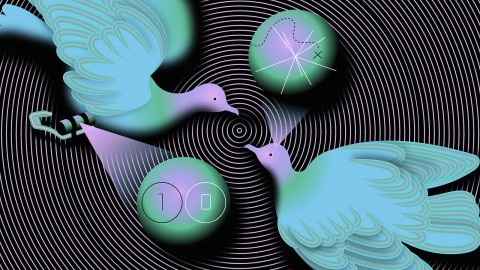
What is quantum information?
Published on 01/18/22
Written by Nathan Collins
Quantum information breaks the rules of classical information in a way that could allow us to answer questions that a classical computer cannot.
Imagine mailing a letter. You, as the person sending the letter, know what the letter says. But the situation is different for the person you’re mailing the letter to. Until they read it, they generally won’t know what it says.
This is the way scientists think about information, at least in the classical sense.
A computer stores information, sends and receives information, and processes information. In a classical computer, the information travels in the form of a string of bits—a pattern of 1s and 0s. As each bit arrives, the recipient doesn’t know what value it will have; from their point of view, it is just as likely to be a 0 as it is to be a 1. To be sure, it will definitely be one or the other, but which it is will be revealed only once it arrives.
In this sense, upon its arrival each bit resolves a certain amount of uncertainty.
Now, it could be that knowing the start of a message gives clues about the rest of it. If a message starts, “O Romeo, Romeo,” it’s a good bet the message will conclude, “wherefore art thou Romeo?”
Still, knowing the first part of the message does not determine—perhaps more to the point, it does not affect—the next part of the message. It could be that the rest of the message is “could you get me a sandwich?”
All of this makes sense because classical information follows a set of rules.
Quantum information breaks those rules, making it at once a powerful basis for computing and an exquisitely fragile beast.
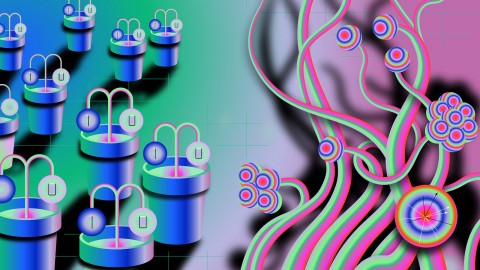
From bits to qubits
Published on 01/13/22
Written by Sarah Charley
Quantum computers go beyond the binary.
The first desktop computer was invented in the 1960s. But computing technology has been around for centuries, says Irfan Siddiqi, director of the Quantum Nanoelectronics Laboratory at the University of California, Berkeley.
“An abacus is an ancient computer,” he says. “The materials science revolution made bits smaller, but the fundamental architecture hasn’t changed.”
Both modern computers and abaci use basic units of information that have two possible states. In a classical computer, a binary digit (called a bit) is a 1 or a 0, represented by on-off switches in the hardware. On an abacus, a sliding bead can also be thought of as being “on” or “off,” based on its position (left or right on an abacus with horizontal rods, or up or down on an abacus with vertical ones). Bits and beads can form patterns that represent other numbers and, in the case of computers, letters and symbols.
But what if there were even more possibilities? What if the beads of an abacus could sit in between two positions? What if the switches in a computer could consult each other before outputting a calculation?
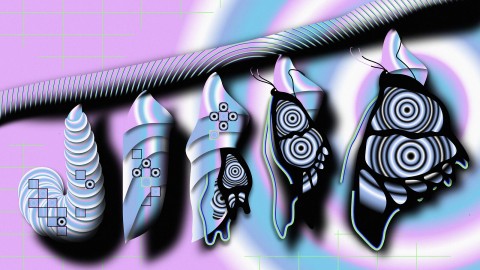
The second quantum revolution
Published on 01/12/22
Written by Daniel Garisto
Inventions like the transistor and laser changed the world. What changes will the second quantum revolution bring?
For physicists trying to harness the power of electricity, no tool was more important than the vacuum tube. This lightbulb-like device controlled the flow of electricity and could amplify signals. In the early 20th century, vacuum tubes were used in radios, televisions and long-distance telephone networks.
But vacuum tubes had significant drawbacks: They generated heat; they were bulky; and they had a propensity to burn out. Physicists at Bell Labs, a spin-off of AT&T, were interested in finding a replacement.
Applying their knowledge of quantum mechanics—specifically how electrons flowed between materials with electrical conductivity—they found a way to mimic the function of vacuum tubes without those shortcomings.
They had invented the transistor. At the time, the invention did not grace the front page of any major news publications. Even the scientists themselves couldn’t have appreciated just how important their device would be.
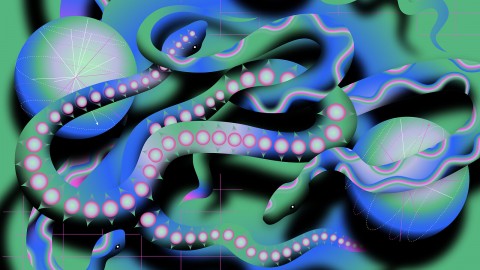
Playing by the quantum rules
Published on 01/11/22
Written by Nathan Collins
Uncertainty, entanglement, spooky action: On the quantum scale, the universe doesn’t work the way you might expect.
While driving down the highway, physicist Werner Heisenberg is stopped by a police officer, the physics joke begins.
“Do you know how fast you were going?” the officer demands.
When Heisenberg shakes his head, the officer tells him: “You were doing 90.”
“Great,” Heisenberg complains. “Now I don’t know where I am.”
To get the joke, you need to be familiar with the Heisenberg uncertainty principle, Heisenberg’s observation that it’s impossible to simultaneously measure both the velocity and position of certain objects.
It’s a joke because, of course, the uncertainty principle does not apply to something the size of a person or a car.
The uncertainty principle comes from quantum physics, which deals with much smaller objects—things like atoms and quarks. The quantum world differs from the classical world we’re used to in a variety of ways.
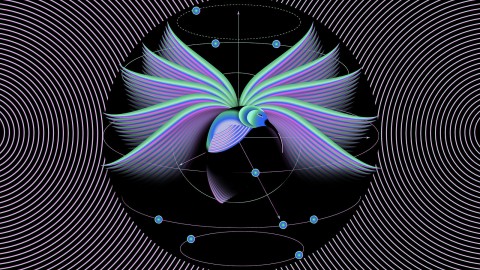
Looking at a new quantum revolution
Published on 01/11/22
Written by Kathryn Jepsen
This month (January 2022), Symmetry presents a series of articles on the past, present and future of quantum research—and its many connections to particle physics, astrophysics and computing.
On July 25, 2018, a group of scientists from Microsoft, Google and IBM sat on a stage at the Computer History Museum in Mountain View, California. Matthias Troyer, John Martinis and Pat Gumann were all working on research into quantum computing, which takes advantage of our knowledge of quantum mechanics, the physics of how the world operates at the smallest level.
The evening was billed as a night to ask the experts “Quantum Questions.”
About an hour into the event, moderator and historian David Brock asked the scientists one last thing: “What do you think—for us as, you know, citizens of the world—what are the most important things for us to know about and keep in mind about quantum computing, as it is today?”